Abstract
The skeletal remains of two past British populations; Baldock, Hertfordshire and St. James Abbey, Northamptonshire were analysed using osteological macroscopic techniques to determine the relationship between Schmorl’s nodes, body mass and stature. Results indicate that there is no statistical correlation (r=0.010) between the true prevalence rate (TPR) of Schmorl’s nodes and body mass in the Baldock population, and only a weak correlation (r=0.224) observed in the St. James population, although this was considered statistically insignificant (p=0.282). When all individuals were analysed together a statistically significant (p=0.003) correlation (r=0.444) was determined between stature and Schmorl’s nodes prevalence (frequency). The greatest frequency of Schmorl’s nodes was observed on the lower thoracic and upper lumbar spine, which confers with several previous studies. The implications of Schmorl’s nodes, body mass and stature have been discussed within the context of health and lifestyle, and differential aetiologies have been explored for the manifestation of the pathology. The authors hypothesise that multiple aetiologies likely contribute to Schmorl’s nodes formation, with aetiology being influenced by several factors e.g. age, occupation, and lifestyle. However, the evidence suggests axial loading of the spine likely represents the greatest single influence on node formation across populations.
Introduction
Schmorl’s nodes are depressions on the superior and/or inferior surfaces of the vertebral body (figure 1), due to herniation of the nucleus pulpous through the cartilaginous end plate. They are most common on the lower thoracic (T8-T12) and the upper lumbar vertebrae (L1-L2) (Rogers and Waldron 1995, 27). Schmorl’s nodes are of significant interest in bioarchaeology and have been used to interpret past human health and lifestyle and offer an indication of trauma within a historical population (Üstündağ et al. 2010; Novak et al. 2012). Clinically they are frequently asymptomatic but can cause acute backpain, and thus present a problem in modern populations (Kyere et al. 2012, 2119; Abu-Ghanem et al. 2013). The reported clinical and palaeopathological literature indicates that Schmorl’s nodes prevalence ranges vastly between 5 and 70% (Silberstein et al. 1999, Dar et al. 2010, 672). Comparison between historical populations is difficult due to variation in the methodological approach of assessing Schmorl’s nodes, in addition to a limited understanding of the aetiology of the condition (Matt and Rehman et al. 2014; Trzciński et al. 2017, 301-303). Clinically, magnetic resonance imaging (MRI) (figure 2) is used to assess the number and severity of Schmorl’s nodes in living individuals (Mattei and Rehman 2014, 39). In osteoarchaeology, the pathology is often identified and assessed during post-excavation analysis of past populations via macroscopic techniques.
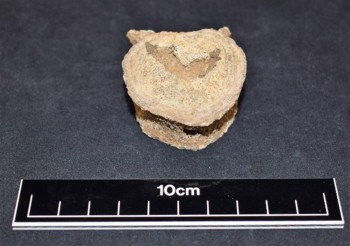
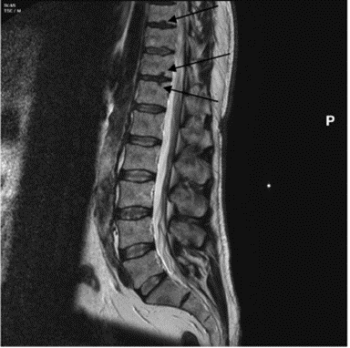
The aetiology of Schmorl’s nodes is still largely unknown, with most osteoarchaeological studies remaining inconclusive in their results (e.g. Saluja et al. 1986). Schmorl’s nodes are commonly thought to relate to physical stress and trauma (Roberts and Manchester 2010, 139), with the manifestation of the depression often being considered a result of high axial loading of the spine (Wagner et al. 2000; Baranto et al. 2006; Nagashima et al. 2013). Other studies suggest a link between development of the foetus and Schmorl’s nodes (e.g. Chan et al. 2014, 90). Alternatively, Schmorl’s and Junghanns (1971) have suggested the vertebral blood vessel process could lead to persistence of vascular channels in the cartilaginous end plate, therefore resulting in herniation of the nucleus pulposus and the development of the pathology. A more recent hypothesis links shape and size of vertebral body, size of neural foramen, and shape of the superior articular facets and pedicles with Schmorl’s nodes severity (Plomp et al. 2012, 2015), whilst Williams et al. (2007) has proposed a genetic aetiology for the pathology. Furthermore, the relationship between Schmorl’s nodes and age is inconclusive across both clinical (Hilton et al. 1976; Abbas et.al 2018) and osteoarchaeological studies (Jiménez‐Brobeil et al. 2010; Üstündag 2009, 700), and such a broad range of results indicates Schmorl’s nodes could have a multifactorial aetiology.
The relationship between Schmorl’s nodes, body mass, and stature is less understood, and has undergone little research in the palaeopathological literature. Body mass and stature are the result of both environmental and genetic factors (Maes et al. 1997; Silventoinen et al. 2000), and therefore must be considered as possible aetiologies for Schmorl’s nodes. It has been hypothesised that increasing body weight and stature have a linear effect on spinal loading (Han et al. 2013; Hajihosseinali 2015, 276), and it is expected this should correlate with Schmorl’s nodes prevalence in the spine. A recent clinical study conducted by Sheng et al. (2017) analysed 23,048 individuals from the US 2014 medical panel study, determining a positive correlation between obesity and intervertebral disc problems, whilst other studies have highlighted a relationship between spinal degeneration (including Schmorl’s nodes manifestation) and obesity (Samartzis et al. 2012). Despite this, the understanding of the relationship in osteoarchaeological contexts is poorly understood, and has undergone limited research, with Trzciński et al. (2017) reflecting the only significant study to assess the relationship between Schmorl’s nodes, stature and body mass, concluding a statistically significant correlation between all variables under analysis.
This study aims to build upon this work and investigate whether body mass and stature affects the manifestation of Schmorl’s nodes in the St. James and Baldock skeletal populations.
Materials and Methods
Populations
The cemetery associated with St. James Abbey was excavated in the winter of 2000-2001 (Northamptonshire Archaeology, nd), with nearly 300 skeletons being exhumed. The recorded burials included 240 men and forty women, some of whom were of high status, dating to between the twelfth and fifteenth centuries (Serjeantson and Adkins 1906).
The skeletal remains from the Land to the Rear of Baldock, California date to between the first century BC and second century AD. The 57 skeletons were recovered in the 2010-2011 excavations, consisting of 46 adults, from which 25 were males and 18 females (Keefe et al. 2015, 1).
Both skeletal assemblages are currently housed at PalaeoHub, Department of Archaeology, University of York.
Sample selection
A specific criterion of adult male individuals was required for the study, as clinical and osteoarchaeological literature have indicated this age and sex demographic typically display the highest prevalence for the pathology (Dar et al. 2009; Haidar et al. 2010, 19). 44 skeletons were deemed suitable for analysis based on sex, age, and completeness of vital osseous elements (spinal vertebrae and proximal femur).
Assessment of Age and Sex
Age and sex were obtained from the original records, but both were reanalysed to ensure the parameters of the study were met, in addition to maintaining the accuracy of information on the skeletal remains. Both the pubis symphysis (Brooks and Suchey 1990) and auricular surface (Buikstra and Ubelaker 1994) were used to determine age. Skeletons were allocated into four age categories according to the system outlined by Cox (2000): young adult (YA) (18-25), young middle adult (YMA) (26-35), old middle adult (OMA) (36-45), and mature adult (MA) (46+). Skeletons assessed to be younger than eighteen were not used in this study in accordance with the assessment criteria outlined above.
Sex was determined in accordance with standard guidelines for recording human remains (Brickley & McKinley 2004; Cox & Mays 2006; Buikstra and Ubelaker 1994), with skeletal sex indicators from both the skull and pelvis being examined. All individuals analysed were determined to be male which confers with the previous assessment.
Assessment of Schmorl’s Nodes
The thoracic and lumbar spine of 44 skeletons was analysed for the macroscopic presence of Schmorl’s nodes. The cervical vertebrae were not analysed as the lesions rarely develop in this region of the spine (Roberts and Manchester 2010). The number of vertebrae present for each skeleton analysed was first determined, followed by the morphological assessment of Schmorl’s nodes on the superior and/or inferior vertebral surfaces, and characterised by a depression with a sclerotic margin (figure 3). The condition was counted once per a vertebra. The system of recording was influenced by the York Osteoarchaeology Ltd and implemented uniformly across the study. The crude prevalence rates (CPR), and true prevalence rates (TPR) of Schmorl’s nodes (Waldron 2017) were calculated using the following equations:
Additionally, the frequencies of Schmorl’s nodes for both the superior and inferior vertebral bodies was calculated for the different spinal regions: upper thoracic (T1-T6), lower thoracic (T7-T12), and lumbar spine.
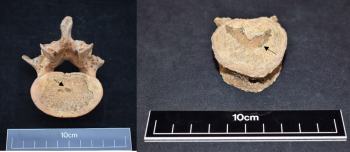
Stature estimations
The previously recorded stature data for 44 skeletons was accessed from the original records and used to determine the statistical difference between the Baldock and St. James populations and to establish if a correlation existed between Schmorl’s nodes and stature. The stature was based on complete long bone length and calculated following Trotter and Gleser (1958) in the Baldock population and Trotter (1970) in the St. James population.
Body mass estimation measurements
The left femur (figure 4) when possible was used to take the proximal femoral head measurement to estimate body mass in accordance with Ruff et. al (1991). To ensure accuracy and precision, an electronic calliper was used with a range of 150mm, and precision of 0.1mm. Individuals displaying gross destruction of the proximal femoral head region affecting measurements were not included in the study. In some cases, the left femur was not available for measurement due to absence or poor preservation, hence the right femur was measured instead, having a limited effect on the calculation of body mass (Auerbach and Ruff 2006).
The femur was chosen to determine body mass because of the relative completeness throughout both populations of the osseous element. The use of the pelvis to ascertain body mass (Ruff 2000) was not possible due to widespread pelvic incompleteness.
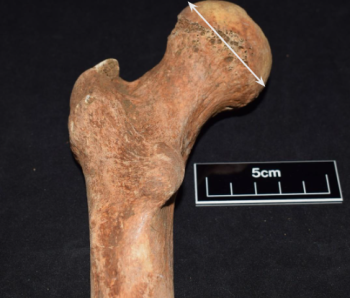
To calculate body mass the formula outlined by Ruff et al. (1991) was used:
Weight (kg)=[(2.741× femoral head bredth (mm))-54.9]×0.9Statistical Analysis
Statistical analysis was performed using the software SPSS version 26 to determine the statistical difference between populations and the correlation between the variables analysed (Khamis 2008, 157-159) (table 1 and 2). The numerical values for correlation ranged from +1.0 to -1.0, and the numbers indicate both the strength and direction of the correlation. It is accepted that greater than 0 indicates a positive correlation (as one variable increases so does the other and vice versa) and less than 0 indicates a negative correlation (as one variable increases the other decreases, and vice versa). The closer the coefficients are to +1.0 or -1.0, the stronger the relationship between the two variables is. The statistical significance for all variables correlated was also determined, with a significance level below 0.05 (less than 5%) indicating a significant correlation.


Results
Overview
The data for 44 skeletons was analysed and interpreted and is presented in graph and table format (table 3).
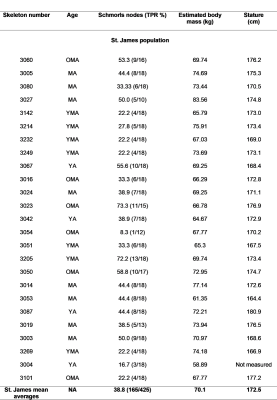
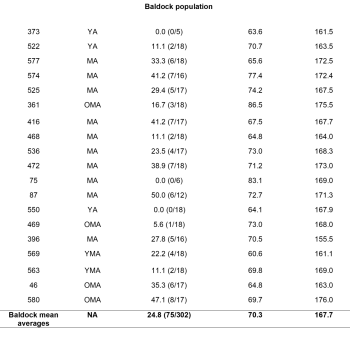
Schmorl’s nodes
In the St. James population, there were 425 vertebrae available for analysis, 285 thoracic, 118 lumbar, and 22 sacral. The Baldock population had 302 vertebrae, 202 thoracic, 82 lumbar, and 18 sacral. A greater TPR was present in the St. James population at 38.8% (165/425) compared to 24.8% (75/302) in Baldock (figure 5), with this being determined as statistically significantly different (p=0.006)
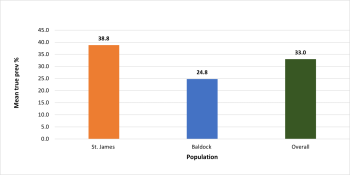
Despite a contrasting TPR of Schmorl’s between the populations a similar distribution was observed within the spine. In the St. James population, the most frequently affected vertebrae were between T6 and L2, with the peak prevalence occurring on T9, T10 and T11. A similar pattern of distribution was observed in the Baldock population with the most affected vertebrae located between T6 and L1, with the peak on T8 and T9. In both populations T1 and S1 were not affected (figure 6).
The inferior lower thoracic spine was more commonly affected by the pathology compared to other spinal regions (table 4).
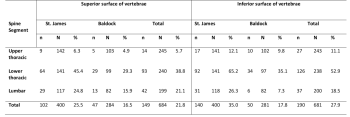
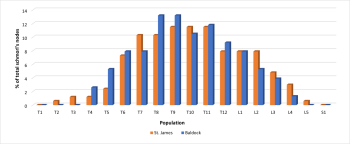
Body mass
There was no statistically significant difference (p=0.751) between mean body mass in the St. James (70.1kg) and Baldock (70.3kg) populations (figure 7).
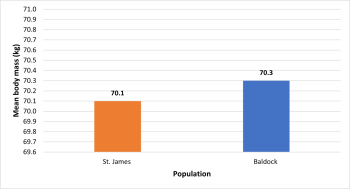
Stature estimation data
The male stature in St. James and Baldock populations (figure 8) was determined to be statistically significantly different (p= 0.001).
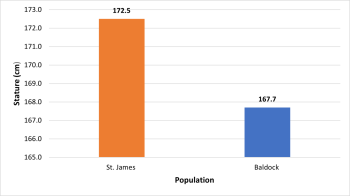
Schmorl’s nodes and age
The TPR of Schmorl’s nodes was 38.9% (28/72) in YA, 31.7% (40/126) in YMA, and 42.7% (56/131) in MA respectively (figure 9). Therefore, statistical analysis indicated a weak correlation (r= 0.224) between Schmorl’s nodes and age in the St. James population, but this was determined to be insignificant (p= 0.122).
In the Baldock population, two YA and one MA, were observed with no macroscopic evidence of Schmorl’s nodes. The highest TPR was observed in the MA category with 31.6% (49/155) of the vertebrae being affected. A statistically significant (p= 0.025) positive correlation (r=0.424) indicating an increase in Schmorl’s nodes with age was determined in the Baldock population.
When the populations were analysed together a statistically significant (p= 0.032) positive correlation (r=0.255) was determined between Schmorl’s nodes prevalence and age.
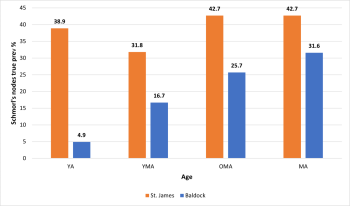
Schmorl’s nodes and body mass
In the St. James population, a weak statistical correlation (r=0.224) was determined between Schmorl’s nodes and body mass, indicating that an increase in body mass was associated with an increase in Schmorl’s nodes prevalence, although this was not deemed statistically significant (p=0.282).
A statistical correlation (r= -0.010) was not identified in the Baldock population between Schmorl’s nodes and body mass variables. When the results were pulled for both population samples a very weak positive correlation was observed (r=0.076), but this was not significant (p=0.623).
More in depth analysis revealed no statistical correlation between Schmorl’s nodes frequency and regions of the spine, or between frequency and superior and inferior surfaces (see table 5). However, in the St. James population a positive correlation (r=0.396) with near statistical significance (p= 0.050) was observed in the superior lumbar spine, indicating an association between body mass, and increasing Schmorl’s nodes frequency.
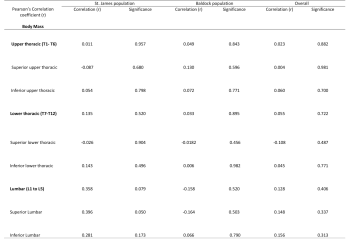
Schmorl’s nodes and stature
When both the Baldock and St. James populations were analysed together a significant (p=0.003) positive correlation (r=0.444) was determined between Schmorl’s nodes and stature. However, when the populations were analysed individually a correlation was identified but this was not deemed to be statistically significant.
More in depth analysis revealed a statistically significant positive correlation between Schmorl’s nodes frequency and stature in the upper thoracic (superior and inferior) and lower thoracic (inferior), but not the lumbar spine (see table 6).
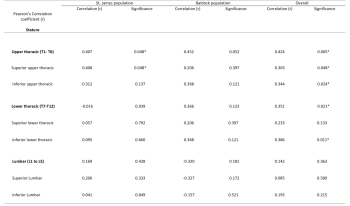
Discussion
This study found a statistical correlation between Schmorl’s nodes and estimated stature, but no correlation between Schmorl’s nodes and estimated body mass. A higher frequency of Schmorl’s nodes was also established on the lower thoracic inferior spine, which confers with several previous studies (Pfirrmann and Resnick 2001, 368; Dar et al. 2010, 673). However, overall, it is not clear whether Schmorl’s nodes are directly correlated with either body mass or stature, and hence the effect of axial loading on the spine on Schmorl’s nodes development remains obscure in both the clinical and indeed the palaeopathological literature. As in other studies, the relationship between Schmorl’s nodes formation and age was unclear (Hilton et al. 1976; Abbas et al. 2018), but when the population samples were pulled a significant (p=0.032) positive correlation (r=0.255) was observed, suggesting increasing prevalence with degenerative age.
The palaeopathological and clinical literature has suggested a higher body mass increases the force applied to intervertebral discs in the lower spinal region (Liuke et al. 2005, 903; Trzciński et al. 2017, 308; Sheng et al. 2017, 5) and that Schmorl’s nodes are largely associated with the lower thoracic and lumbar spine due to increased axial loading (Dar et al. 2010). Trzciński et al. (2017) established a relationship between body mass and Schmorl’s nodes frequency, and here the authors suggested body mass likely increased axial loading on the spine in the populations under analysis. In both Baldock and St. James Schmorl’s nodes did not correlate with body mass, suggesting this was not a significant influence on the aetiology in these populations. Despite this, the high frequency of Schmorl’s nodes in the lower thoracic and lumbar vertebrae does suggest an aetiology linked to high axial loading of the spine (Burke 2012, 6; Dar et al. 2010, 674). It should be acknowledged that only a small sample population was analysed from Baldock (n=19) and St. James (n=25) and to establish accurate results for the relationship between body mass and Schmorl’s nodes development all available skeletons from these populations (males, females, and non-adults) should be analysed in the future.
The influence of stature on Schmorl’s nodes manifestation has been largely unexplored in the clinical and palaeopathological literature. This study established a statistically significant correlation between stature and Schmorl’s nodes frequency (when samples were pulled), and this was also observed by Trzciński et al. (2017). This could reflect the greater vertebrae size associated with taller individuals, and therefore a lower ratio of vertebral body to intervertebral disc would be observed offering less protection against axial forces, and predisposing individuals to herniation of the nucleus pulpous (Dar et al. 2010; Plomp 2015; Trzciński et al. 2017, 307).
The loading of the spine is not exclusively affected by stature and body mass, and sudden bending, stretching and rotating movements characterised by lifting objects off the ground would cause increased loading on the spine, possibly resulting in trauma (Kyere et al. 2012, 2116-2117). Therefore, it is important to consider the occupation of the populations under analysis. St. James Abbey was primarily occupied by monks and historical documentation indicates a largely sedentary lifestyle for these individuals (Knüsel et al. 1997, 482). The population also included lay individuals, and possibly benefactors and lay brothers working for the clergy and the monks. It can also be inferred from the different burial statuses that some individuals interred in the St. James cemetery were likely hired to maintain the Abbey and perform manual labour tasks, although with no documentation for individual burials this remains a theory. It might explain why a high prevalence of Schmorl’s nodes was observed in young adult individuals (38.7%), and these men may reflect a largely peasant population that were responsible for maintaining the Abbey. Similarly, both clinical and palaeopathological studies have associated early development of Schmorl’s nodes with high axial loading relating to occupation and lifestyle (Cooper 1996, 42; Rachbauer et al. 2001). Stirland and Waldron (1997, 334) analysed the immature skeletons from the crew of the Mary Rose, and then compared these individuals to the contemporary Norwich cemetery of St Margaret Fyebridgegate. It was determined that a high frequency of Schmorl’s nodes in the Mary Rose individuals likely reflected manual labour from a young age. Alternatively, these young adults could be practicing monks (or individuals studying to be monks) and future research on the St. James population would benefit from analysing the skeletal material within a social burial context. This could reveal patterns of pathology associated with different burial types.
Nothing is known of the occupation or social status of the Baldock population males, and thus it is difficult to determine factors other than age, body mass and stature that influenced high axial loading on the spine. The town of Baldock functioned as a sub-regional marketplace for small craft production, although it is evident from osteological assessment that a large proportion of the population were agricultural labourers (Fitzpatrick-Matthews 2007, 153-154; Fitzpatrick-Matthews 2016, 35). It is therefore reasonable to assume that strenuous lifting and agricultural work from a young age would have applied considerable axial stress to the spine of the Baldock males (Cooper 1996, 42).
It must be acknowledged that loading of the spine does not exclusively explain the pattern of Schmorl’s nodes distribution or its aetiology. This study, as in many others (Saluja et al. 1986, 93; Dar et al. 2010), indicates a high frequency of Schmorl’s nodes in the lower thoracic and upper lumbar spine. However, if loading of the spine was the only cause of the pathology then the highest frequency would be expected in the L5 vertebrae, but this is not the case, and frequency commonly decreases after L2 (Dar et al. 2010).
Other factors, such as congenital, spinal and metabolic diseases, in addition to trauma, abnormal curvature of the spine, and even the shape of vertebrae can influence the manifestation of Schmorl’s nodes (Hilton et al. 1976; Wagner et al. 2000; Williams 2007, 855; Skaf et al. 2011; Kyere et al. 2012; Plomp 2015), and future work must consider a multi-factorial approach to assessing Schmorl’s nodes aetiology (see figure 10). Therefore, the broad variation in results obtained from both clinical and archaeological studies suggest aetiology could vary considerably depending on the population, and the current authors (this article) emphasise the need to consider factors that could be influential in Schmorl’s nodes manifestation, such as: age, sex, diet, socioeconomic status, occupation, and environment (Roberts and Manchester 2010, 43). Despite this, considering the high frequencies for Schmorl’s nodes and their analogous distribution in the spine between different populations, axial loading likely represents the greatest single influence on node formation (Kyere et al. 2012, 2119). In the future, standardisation of recording Schmorl’s nodes in the axial spine, and consideration of node size, location and severity may be able to shed light on inter and intra population level aetiologies for the pathology.
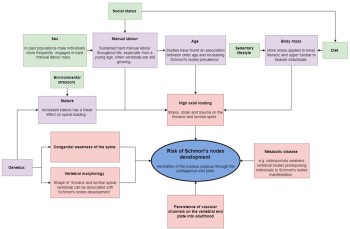
Limitations
When analysing the Baldock and St. James populations, it became clear that many of the vertebrae were poorly preserved, making identification of specific vertebrae difficult. This was a particular problem in the Baldock population, where 47.4% (9/19) of the adult males analysed were determined to have been affected by poor preservation of bone. Furthermore, the actual classification of Schmorl’s nodes was difficult on occasion due to severe degradation to the vertebral surfaces, and this could have potentially caused a low count for Schmorl’s nodes in the Baldock population. This study also suffered from a small sample size, and it is difficult to assess how representative the skeletal remains were of both the Baldock and St. James population. Indeed, Baldock had a disproportionate number of older adults, with prime adults (18-36) being considerably underrepresented. It is not known whether this is truly reflective of the population, or due to sampling bias.
Conclusion
This study could not determine the exact aetiology of Schmorl’s nodes through the assessment of body mass and stature. It is evident though that both the Baldock and St. James populations displayed no observable statistical correlation between the prevalence of Schmorl’s nodes and body mass. Despite this, a statistically significant correlation was observed between Schmorl’s nodes and stature (when populations pulled) which supports an axial loading hypothesis as the primary cause of Schmorl’s nodes formation in past and present populations. Finally, it should be acknowledged that to determine the most probable aetiology for Schmorl’s nodes, all environmental and genetic factors should be considered. Additionally, a standard system of recording should be used that assesses node size, shape of node, severity, node location, vertebrae size, vertebrae shape, and other palaeopathological indicators that could have influenced Schmorl’s nodes formation e.g. osteoporosis and underlying congenital weaknesses of the spine.
Acknowledgements
The authors would like to thank the Heritage Network Ltd and Northamptonshire commercial archaeology unit for the skeletal remains from Baldock and St. James analysed in this article. Additionally, we would like to thank Malin Holst for her advice and support.